RADIOMETRIC DATING METHODS
Based on
Critique of Radiometric Dating
by Harold S. Slusher
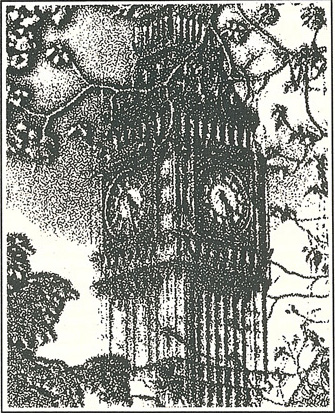
adapted from an article by
D.B. Gower
D.Sc., Ph.D., C. Chem.
Used with the kind permission of the Creation Science Movement, 50 Brecon Avenue, Cosham, Portsmouth, England, P06 2AW.
A variety of geochronometers, or “earth clocks,” have been consulted in the centuries-old quest to measure the age of the earth. Techniques as diverse as population statistics, tree-ring counting (dendrochronology), magnetic-field analysis, and several different methods of radiometric dating have been employed in the effort to gauge the passage of geological time. Unfortunately, one of the problems of geochronology is that the various methods of dating have given vastly different values for the age of the earth: the salt-in-sea-water approach gives 50 to 90 million years; the analysis of helium gas in the atmosphere suggests an age of 26 million years; and the helium content of meteorites yields a figure of about 60 million years, even though Potassium-Argon analysises on these space visitors lead scientists to believe the earth is at least 4,600 million (i.e., 4.6 billion) years old.
This article will examine the reliability of radiometric methods of dating, often perceived by the layman to be accurate and unassailable.
THE PHYSICS OF RADIOACTIVITY
Matter is made up of molecules, which are in turn comprised of atoms. The overall configuration of the atom is rather like a miniature planetary system. At the core of every atom is a nucleus containing at least one positively charged proton and, with the exception of hydrogen, at least one neutron (no charge). Atomac nuclei are surrounded by negatively charged electrons. The number of electrons governs the chemical reactivity of the element, and the number of protons in an atom generally equals the number of electrons. (If an atom is ionized, one or more electrons will be missing.)
An atom may or may not have the same number of neutrons as it does protons. Two atoms having the same number of protons but a different number of neutrons are called isotopes of that particular type of atom. Isotopes display similar chemical properties because they have the same number of protons and electrons. Many isotopes undergo radioactive decay; that is, they spontaneously convert into chemically different elements by emitting alpha-particles (viz., 2 protons + 2 neutrons - a helium nucleus) or beta-particles (electrons - thereby changing neutrons into protons), often generating gamma-rays (high-energy electromagnetic radiation) in the process.
RADIOMETRIC DATING
For any given isotope, a “decay curve” can be drawn showing the time it takes for half of an isotope to complete the conversion process that accompanies radioactive decay. Examples of the half-lives of some isotopes used for radiometric dating are: Tritium, 12.3 years; 14Carbon, 5,730 years; 40Potassium, 1,300 million years; and 238Uranium, 4,510 million years.
These estimates of time may be considered reliable for use in geochronometry if all of the requirements for a geological clock are satisfied. There are at least five requirements for any chronometer. First, the clock must be sensitive enough to measure the intervals of time involved. Second, there must be a measurable change in some quantity over time. Third, the clock must always run at the same rate (or change at a known rate). Fourth, one must know the starting point of the clock (e.g., the initial quantity of whatever is being measured). And fifth, the clock must never have been “reset” or otherwise interfered with by external influences. Therefore, the radioactive elements in rocks provide a means of dating the rocks:
- if the decay rate is sufficiently slow to measure long intervals of time - if the passage of millions of years is to be clocked - or sufficiently fast to gauge short intervals - if periods of only a few thousand years need to be detected;
- if the amounts of radioactive material and radiogenic elements (products of the radioactive decay process) in the sample can be measured;
- if the half-life of the radioactive material is known and has been constant throughout time;
- if the initial amounts of radioactive substance and its radiogenic elements can be determined; and
- if the measured amounts of radiogenic elements are derived entirely from the decay process, with none having been added or taken away during the span of time being measured.
The first two of these criteria are satisfied with the selection of appropriate isotopes for analysis, the selection being based on current observational data of isotopic behavior. But, as we shall see, the assumptions regarding the starting conditions for radiometric clocks, the constancy of radiometric timekeeping, and the closed-system nature of radiometric geochronometers in situ are poorly founded.
SOME CRITICISMS OF RADIOMETRIC “CLOCKS”
A significant stumbling block for the unqualified acceptance of radiometric dates comes from laboratory evidence indicating that the half-lives of isotopes may not be truly constant, as is generally assumed in radiometric dating. Experiments have shown that half-lives may vary with changes in pressure, temperature, electrical potential, chemical state, etc. (H.D. Dudley, 1976), changes that do in fact occur in the natural world. For instance, the decay rate of 57Iron changed up to three percent under the influence of an electric field (Dudley). Harold Slusher has also observed that when experiments were performed “on molecular monolayers with C14 added (J.L. Anderson, 1971) ... [t]he emitted radiations did not occur in the pattern that classical theory assumes.” If we cannot be certain the half-life of a particular radioactive element in a given sample has not been constant throughout the entire in situ history of the element, and the extent and duration of variations in the decay rate cannot be determined (as in the case in radiometric dating), then the confidence in dates obtained by radiometric methodology is necessarily low. Now let us have a look at some of the problems of particular radiometric dating techniques.
The Uranium-Thorium-Lead Method
The U-Th-Pb method of radiometric dating, in use since 1907, measures the decay of the 238Uranium isotope, which converts to lead and helium. In field samples, it is hard to tell whether or not all of the lead present was produced by the decay of 238Uranium. If lead was present in the initial state, the rock would test much older than it really is. Measuring the amount of helium in the sample could be used to cross-check the amount of decay inferred from lead measurements, but there is good reason to believe that helium in rocks can readily escape into the environment (Holland & Kulp, 1945). One is also faced with the question of how much helium was in the rock to begin with. Therefore, helium measurements are ill-suited for verifying dates obtained from lead measurements in field samples.
Also, it is known that 238Uranium can leach out of rocks, even granite (Hurley). In fact, anywhere up to fifty thousand tons of uranium are washed into the sea annually (Holland & Kulp, 1945). Rock that has lost a portion of its 238Uranium content in this way would appear older by an indeterminate and potentially enormous amount when dated by the Uranium-Thorium-Lead method. And again, as noted above, measuring the amount of extent helium in a sample is of no help in ascertaining the amount of decay that has occurred.
The Uranium-Thorium-Lead method of dating has now been superseded by the Potassium-Argon method.
Potassium-Argon Method
In the Potassium-Argon method of dating, the isotope 40Potassium changes into 40Argon (11%) and 40Calcium (89%). Because radiogenic calcium has the same atomic mass as abundantly common non-radiogenic calcium, it is 40Argon which is used for age determinations. But, as with the U-T-Pb method, there are several factors that get in the way of unreserved acceptance of K-Ar test results.
The first problem centres on the uncertainty of the so-called branching ratio of K40 into Ar40 and Ca40 (Cook, 1966). Although various branching values for Ar40/Ca40 have been used in dating, the arbitrary value of 0.08 is often employed to force agreement with results from the U-Th-Pb method. This branching ratio is low in comparison to the observed ratios of 0.11 to 0.125 and yields artificially high estimates of age for the host rock.
Another problem is that the amount of 40Argon in the earth's atmosphere is 100 times too high for all of it to have been produced by the radioactive decay of 40Potassium over 4.5 billion years (the alleged age of the earth). Either large amounts of 40Argon have been derived from another source or, more likely, 40Argon was present when the earliest rocks were formed.
The assumption of closed-system radiometric decay is violated by the fact that, like helium, 40Argon gas is known to diffuse easily in rock. The rate of diffusion depends on the porosity of the rock and on the local pressure. Rocks deep in the earth's crust (where the pressure from overburden is high) will lose 40Argon to rocks nearer the surface (where the pressure from overlying rock is low). Rocks near the surface - the ones used for dating - can be expected to have a higher 40Argon content than would come from local radioactive decay alone, falsely suggesting great age.
Moreover, field research indicates that volcanic rocks very likely acquire 40Argon from their environment while still in a molten state. Sample lavas taken from the ocean bed near Hawaii in 1968 were assigned dates of up to 22 million years by the Potassium-Argon method, even though the flow is known to have occurred less than 200 years ago! This is not surprising since 40Argon is common in both the earth's crust and the atmosphere. As 40Argon migrates through rock, it tends to congregate in the fissures and cracks used by magma to reach the surface. To the extent volcanic rock incorporates 40Argon picked up from its surroundings, 40Argon dating will incorrectly suggest the passage of great spans of time, because it is assumed the (artificially) high amount of 40Argon present came from the decay of 40Potassium.
As if the K-Ar method did not have enough problems, potassium easily washes out of minerals, just as uranium does. In one test, a meteorite lost eighty percent of its potassium when distilled water was run over it for just 4.5 hours. Therefore, rocks subjected to weathering or in contact with ground water could be expected to lose a portion of their potassium content and give the misleading impression in K-Ar tests that 40Potassium had vast ages in which to decay to a very small quantity.
Rubidium-Strontium Method
The Rubidium-Strontium method is used in trying to date metamorphic rocks. The precise half-life of 87Rubidium is uncertain, but is generally taken to be about 49.9 billion years.
In the same way there is too much 40Argon in the atmosphere to have all come from the decay of 40Potassium, the earth's crust contains ten times more 87Strontium than one would expect to find if it had all been produced by the decay of 87Rubidium. To get around this difficulty, the estimated initial quantity of 87Strontium is usually manipulated to agree with dates given by the U-Th-Pb method.
The possibility of radioactive and radiogenic elements diffusing in the host rock tends to undermine confidence in dates given by this approach, as it does with other radiometric dating methods. Different minerals exhibit different diffusion rates such that even individual grains from the same rock yield dissimilar ages. Whenever the radioactive and radiogenic elements being measured can penetrate or leach out of a given sample of rock, the accuracy of the radiometric method being used becomes suspect. Add to this the fact that 87Strontium can disappear from a portion of rock by ion-exchange processes and the possibility that there may be a considerable isotopic variation in rocks when they are formed, one really has a problem in obtaining dates that warrant a high level of confidence. No matter what efforts are made to overcome the problem of diffusion, one can never be sure that the “corrections” are correct.
The 14Carbon Dating Method
The radiocarbon method of dating was introduced in 1948 as a way of dating organic remains of relatively recent origin. The method has been used extensively in determining the age of archaeological finds. Radioactive 14Carbon is the long-lived isotope of common 12Carbon, it's half-life being approximately 5,730 years. The conversion of 14Nitrogen into 14Carbon is a secondary effect of cosmic radiation bombarding atoms in the upper atmosphere. The radioactive carbon thus formed combines with oxygen to form carbon dioxide. During photosynthesis, plants absorb C14O2 and C12O2 in proportion to their mix in the air. Animals than acquire C14O2 when they eat plants or, in the case of meat-eaters, when they dine on herbivores. Upon the death of an organism, the amount of C14 and C12 is said to be “fixed”; that is, no more carbon will be incorporated into the structure of the plant or animal. At that point, the ratio of C14 to C12 begins to decline as 14Carbon decays into 14Nitrogen. The date assigned to a bone or a piece of wood derives from a comparison of the current1 proportion of C14 to C12 with that which would have existed when the organism died, invoking the half-life of C14 to discover how much time must have elapsed to yield the observed proportion.
As ingenious as this method of dating is, it too involves a number of assumptions. One must assume the following:
- the half-life of 14Carbon has always been the same,
- the rate of 14Carbon formation has always been the same,
- the concentration of carbon in the atmosphere has always been the same,
- the ratio of 14Carbon to 12Carbon has always been constant, and
- the rate of formation and the rate of decay of 14Carbon have always been in equilibrium
The assumption that the amount of cosmic radiation reaching the earth's atmosphere has been constant throughout history cannot be verified, and may not be correct. There are two reasons for saying this. First, it is possible that the earth was once enveloped by a vapour canopy, as suggested by Korff in 1954. If there was such a water vapour canopy before the Flood, and if it was of sufficient density and thickness, it would have shielded the atmosphere from cosmic rays and thereby inhibited the formation of 14Carbon. With less 14Carbon having been present in the antediluvian atmosphere, the rate of accumulation of 14Carbon in living things would have been much less than it is today, making it wrong to infer the passage of vast amounts of time from the small amounts of 14Carbon found in remnants flora and fauna from the pre-Flood world. When living things died and were buried by the Flood, perhaps they simply did not have as much 14Carbon in them as we presume they did because we wrongly assume their atmosphere had the same proportion of C14 to C12 as we have today.
Second, there is reason to believe that the strength of the earth's magnetic field has not been constant throughout the past, but has been decaying. The decay rate has been found to be exponential, with it's half-life estimated to be about 1,400 years (T.G. Barnes, 1971). This means that in less than 10,000 years, the field would have decayed to just one percent of its original value. If true, there would have been more protection against cosmic radiation that further back in time one goes, and hence, progressively less 14Carbon in the air. If unexpectedly low amounts of 14Carbon were incorporated into ancient organisms, the results of radiocarbon dating would be erroneously skewed in favour of older ages.
Regarding the equilibrium of 14Carbon production and decay, it would seem the two processes are not in the equilibrium condition assumed in the radiocarbon dating method. The measured rate of 14Carbon formation exceeds that of 14Carbon decay by at least 24 percent (Cook, 1966). Not only does this pose problems for the steady state model of radiocarbon dating needed to produce reliable dates, it suggests that the earth is less than 30,000 years old - the time needed to bring 14Carbon and 12Carbon into equilibrium.
And, as with other radiometric methods, there is always the possibility that radiometric dates are in error because of foreign material having been incorporated into the sample or native material having been lost in situ.
Therefore, when the assumptions that underlie radiocarbon dating are examined, it becomes apparent that the method can give questionable ages for very old specimens. Recognition of this fact is seen in efforts to calibrate radiocarbon tests using samples of known age (such as wooden artifacts from historical times) or from other methods of dating, such as dendrochronology (which involves the counting of tree rings to gauge the passage of time). Though it is now generally accepted that radiocarbon dating is useful in assigning ages to materials that are up to 10,000 years old, its reliability diminishes quickly when dating anything beyond documented history - back to about 3,000 B.C.
CONCLUSION
Radiometric dating has a number of shortcomings in satisfying the requirements of a reliable geological clock. There is doubt as to whether the radioactive decay rate in any given sample would have always been constant. The starting quantities of radioactive and radiogenic elements cannot be known with certainty, and we are unable to go back in time to analyze the initial state of a sample. It is equally impossible to be sure how much, if any, radioactive or radiogenic material has migrated into or out of a radiometric sample. And, in the case radiocarbon dating, it is possible that the formation of 14Carbon has not always been the same as it is today. Any one of these factors should be enough to cause radiometric dates to be treated with caution. But taken together, the belief that radiometric dating is a reliable geochronometer would seem to be poorly founded.
- Actually, as of 1950, just before nuclear weapons testing artificially changed the C14 to C12 ratio in the atmosphere.
Critique of Radiometric Dating
Harold S. Slusher, M.S., D.Sc.
Institute for Creation Research, San Diego: 1973, 2nd ed. 1981
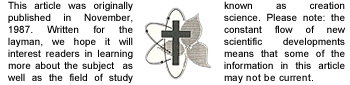